Report 22: Effects of N1-Methyl-Pseudouridine in the Pfizer mRNA Vaccine
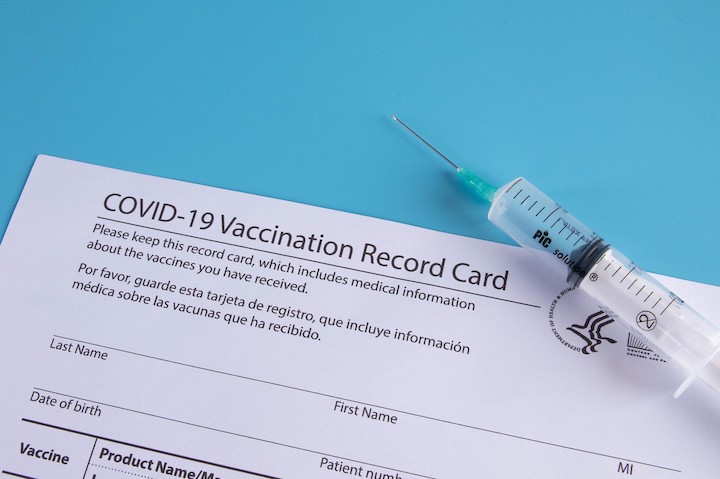
Daily Clout 5/9/22 Report: Effects of N1-methyl-pseudouridine in the Pfizer mRNA Vaccine
Researcher/Author: Daniel B. Demers, PhD
Reviewed by: Team 5
Introduction
The use of messenger RNA (mRNA) vaccines has been developing since 1990. Historically, there have been three significant problems associated with mRNA vaccines. First, it has always been a challenge for vaccine developers to get the desired mRNA into the cells of choice (the delivery problem). Second, introducing a foreign RNA (the vaccine mRNA) into a patient causes their body to initiate an innate immune response thereby causing pathogenesis when there actually was no infection (the immunogenicity problem). And third, RNAs are rapidly degraded by ribonucleases (RNases) which are enzymes that degrade RNA. These RNases are found virtually everywhere which not only hinders development, but also makes it difficult to get a desired mRNA in a vaccine to stay around long enough to elicit the desired response (the degradation problem). There are many summaries of these historical facts (Morais et al., 2021; Jain et al., 2021; Kariko et al., 2008).
The claim among mRNA vaccine manufacturers and some scientists is that the three problems cited above have been solved; but have they?
Both Pfizer and Moderna claim that they solved these problems by encasing the mRNA inside of a lipid nanoparticle (LNP) and by modifying the mRNA through the substitution of N1-methylpseudouridine for the nucleotide uridine (Morais et al., 2021; Jain et al., 2021; Nance and Meir, 2021; Pardi et al., 2018; Andries et al., 2015). The use of LNPs allegedly solves the delivery problem by getting the vaccine’s modified mRNA into the cells and helping to protect the mRNA molecules from degradation during their trip from injection site to target cells. Their use of LNPs is another matter to be addressed in a subsequent report.
The use of a modified uridine (N1-methylpseudouridine) to replace uridine was supposed to solve the last two problems: the inherent immunogenicity of foreign mRNAs and degradation of the mRNA. These matters are the topic of this report.
Does the use of a modified uridine (N1-methylpseudouridine ) solve the problem of the immune response to a foreign RNA such as the vaccine delivered modified mRNA and premature degradation of the vaccine delivered mRNA?
It is difficult to dissect these two issues (mRNA immunogenicity and degradation) because they are so interconnected. But first, what is N1-methylpseudouridine and what does it do?
Modified Uridine
In nature, modified uridines (such as pseudouridine and N1-methylpseudouridine) incorporated into RNA allow the body’s immune system to distinguish “self” from “non-self”; that is, the body’s own RNA molecules (self) from foreign (non-self) RNA molecules (Kariko et al 2005). mRNA-based vaccine development was hindered for years because the body recognized the vaccine mRNA as foreign and initiated an immune response to eliminate the foreign material. Vaccine manufacturers needed a way to suppress that immune response if mRNA vaccines were to be used. But what are the consequences of suppressing the body’s first line of defense, innate immunity?
Pseudouridine was first described in yeast in 1957 (Davis and Allen, 1957) and named the fifth nucleotide, a name it still carries (Borchardt et al., 2020). Pseudouridine is an isomer of uridine; that is, pseudouridine has the same identical atomic composition as uridine but with a slightly different structure. For pseudouridine, although this change is structurally minor, when incorporated into an RNA molecule by the cell in a strategic and specific manner, the changes in properties it imparts to RNA molecules are major.
Besides being involved in gene expression and protein production, natural conversion of uridine to pseudouridine stabilizes the molecule and protects it from degradation by RNases and helps it to evade immune detection (Borchardt et al., 2020).
There is considerable evidence that the use of pseudouridine in vaccine mRNA does in fact protect the mRNA molecule from RNases and thus, slows its degradation and can suppress the unwanted immune response mechanism (Morais et al., 2021; Borchardt et al., 2020; Eyler et al., 2019; Zhao et al., 2018; Kariko et al., 2008). In addition, but not always mentioned, is that pseudouridine increases protein (including spike protein) production (Svitkin et al., 2017). Use of pseudouridine was justified by researchers on the basis that it is a naturally occurring modified nucleotide within our cells and gets strategically and specifically incorporated into many RNA molecules including mRNA. It is known to be involved in multiple aspects of gene expression and protein production (Morais et al., 2021).
However, pseudouridine contributes a universal base character to the nucleotide. Whereas uridine (U) normally base pairs only with adenine (A), pseudouridine exhibits a “wobble” character to it and will allow uridine to base pair with adenine (A), guanine (G), cytosine (C) and uridine (U). These natural modifications in an RNA molecule evidently contribute to its function (Morais et al., 2021; Parr et al., 2020; Svitkin et al., 2017). However, in a vaccine mRNA this would be problematic as it would change the amino acid sequence of the resulting protein; in this case, the spike protein. For a more thorough description of base pairing see the following YouTube video.
The vaccine manufacturers addressed the “wobble” characteristic of pseudouridine by substituting N1-methylpseudouridine into their mRNA construct rather than pseudouridine. N1-methylpseudouridine is different from uridine or pseudouridine but has been shown to demonstrate the beneficial attributes of pseudouridine that the manufacturers sought (protection from degradation, evasion of immune detection, increased protein production, molecule stability) while eliminating the “wobble” character that pseudouridine exhibited (Svitkin et al., 2017; Parr et al., 2020; Morais et al., 2021; Nance et al., 2021).
N1-methylpseudouridine is also naturally occurring but with much lower frequency, and structurally and chemically, it differs considerably from pseudouridine. N1-methylpseudouridine has an added methyl group (CH3) and this modification probably contributes to its higher affinity for pairing with adenine, a much desired attribute for a vaccine mRNA because it is the normal pairing (Morais et al., 2021).
But in nature, modified nucleotides are strategically and specifically inserted and required for proper folding, stability and accurate decoding of RNA molecules (Wurm et al., 2012). Wu et al. (2015) found that abolishing specific pseudouridines in another type of RNA (ribosomal RNA or rRNA) severely affects ribosome function.
Borchardt et al. (2020) used mass spectrometry to analyze mRNA pseudouridine content. They found that pseudouridine was present at 0.2 to 0.6% of total uridine in mRNA from human HEK293T cells (a human immortalized cell line). They hypothesized that mRNA pseudouridylation controls metabolism in response to cellular conditions, and stress conditions induce changes in expression of these modified nucleotides. The placement of pseudouridines affects the RNA backbone conformation and stability of base pairs. Furthermore, pseudouridine alters RNA-protein interactions for several RNA binding proteins (RBPs) that regulate RNA processing. Borchardt et al. (2020) states that “artificial pseudouridylation of a single position can inhibit function.” Furthermore, they state that “pseudouridine is not always treated as a uridine by the ribosome and could affect translation of the protein.”
Therefore, given that the amount of pseudouridine is relatively small in nature (0.2 to 0.6% of total uridine in mRNA), and that the points of pseudouridine insertion are strategic and specific, and that even this amount of pseudouridylation is not well understood, what would be the anticipated outcome of total replacement of a foreign mRNA uridine population with an even more rare modified nucleotide, N1-methylpseudouridine? That is precisely what Pfizer did in its mRNA vaccine. They did not strategically and specifically replace some uridines in their already modified mRNA (already producing two amino acid substitutions in the spike protein), they replaced all uridines in the mRNA (Nance et al., 2021). But this issue is not mentioned in the Pfizer document 2.4 NONCLINICAL OVERVIEW (https://www.phmpt.org/wp-content/uploads/2022/03/125742_S1_M2_24_nonclinical-overview.pdf) or in two papers published as a result of Pfizer’s Phase 1/2 trials (https://www.phmpt.org/wp-content/uploads/2022/04/125742_S1_M5_5351_c4591001-fa-interim-publications.pdf).
The knowledge base of pseudouridine is limited. Borchardt et al. (2020) summarizes it well; “Despite intensive investigation of the structural and biochemical effects of pseudouridine in various systems, the biological role of most endogenous pseudouridine remain unknown.” They continue, “Pseudouridine likely affects multiple facets of mRNA function including reduced immune stimulation by several mechanisms, prolonged half-life, as well as potentially deleterious effects on translation fidelity and efficiency.” Furthermore, the authors stated, “The functions of endogenous pseudouridine in mRNA remain to be discovered.” They go on to state that RNA pseudouridyation could have widespread effects on RNA metabolism and gene expression and that “much remains to be known.”
Given that there is still so much to learn about how endogenous pseudouridine affects biological systems, we must ask ourselves what effects N1-methylpseudouridine might have on these same biological systems, especially considering that so little is known about N1-methylpseudouridine. Afterall, the enzyme, N1-methyltransferase, the enzyme that catalyzes the synthesis of N1-methylpseudouridine, was only identified in 2012 (Wurm et al., 2012). Studies on N1-methylpseudouridine began in earnest in 2015 (Andries et al., 2015). The history of pseudouridine dates back to the 1950s whereas the history of N1-methylpseudouridine only dates back to 2012. Obviously, science has barely scratched the surface of N1-methylpseudouridine and its effects on biological systems.
The incorporation of N1-methylpseudouridine in a mRNA vaccine is obviously not strategic and specific as in natural incorporation. Rather, Pfizer used a shotgun approach, and they had no idea what the ramifications and unintended consequences of such a modification would be. How are the folding, function, localization and clearance of the subsequent protein affected? What does such a massively modified foreign mRNA do to the delicate balance of cells and bodies (homeostasis) that receive it?
To date, there has been nothing identified in nature that resembles the Pfizer modified mRNA, nothing even close. How does this Pfizer modified mRNA interact with the cells’ protein machinery? Where does it localize within the human body? How long does it last? Are there long-term toxicity, carcinogenicity or pharmacological concerns? None of this has been studied. In fact, there is no mention of pseudouridines or N1-methylpseudouridines in the Pfizer document 2.4 NONCLINICAL OVERVIEW (https://phmpt.org/wp-content/uploads/2022/03/125742_S1_M2_24_nonclinical-overview.pdf).
Good science demands answers to these important questions, and the answers should have been obtained before injecting hundreds of millions of people globally (billions of doses) with such an experimental substance.
Immunogenicity: Solution or Problem?
Vertebrates, including humans, have evolved an immune system to eliminate pathogens. That system has two major branches, innate and adaptive immunity. The innate immune system is the body’s first line of defense. Frizinsky et al. (2019) states that it is “more than the first line of defense, it is crucial to the survival of the host.” The body reacts quickly to foreign RNA molecules by producing interferon, cytokines and chemokines (Kang and Compans, 2009; Pardi et al., 2018). These molecules, and others, are released by the cells to protect the body through cell signals and pro-inflammatory responses. They may also impact the adaptive immune response which is the second line of defense. This report will only consider the innate immune response to a modified mRNA invader.
The effects of innate immunity on vaccine mRNA are incompletely understood but there does seem to be agreement that it prevented traditional mRNA vaccines from being used because the foreign RNA gets cleared by the immune system (Kariko et al., 2005; Svitkin et al., 2017; Borchardt et al., 2020; Parr et al., 2020; Morais et al., 2021; Jain et al., 2021). Pardi et al. (2018) noted that some mRNA-based vaccine platforms induce interferon which is associated with inflammation and potentially autoimmunity, edema, blood coagulation and thrombosis. It also increases cytotoxicity leading to apoptosis (cell death) which of course reduces the effectiveness of the vaccine.
Pepini et al. (2017) stated that “activation of the innate immune response by RNA vaccines is potentially a double-edged sword.” On the one hand, with activation of an innate immune response comes release of interferon and cytokines which facilitate the adaptive immune response (which might be needed later). On the other hand, it may, as discussed by Pardi et al. (2017), cause an inflammatory response to the vaccine leading to flu-like symptoms and potentially autoimmunity, edema, blood coagulation and thrombosis, as well as degradation of the vaccine mRNA. As early as February 2020, at that critical time of conception of the Pfizer mRNA construct, it was reported that “the influence of modified bases on the function of a synthetic RNA is poorly understood” (Parr et al., 2020). But it was known that modified RNA, containing pseudouridine or N1-methylpseudouridine, did suppress innate immunity. Aside from helping the vaccine’s modified mRNA to survive in the body, the consequences of suppressing innate immunity simply were not known.
Despite this lack of knowledge involving suppression of the innate immune system, Pfizer still chose to use mRNA modified with N1-methylpseudouridine (Morais et al., 2021; Nance et al., 2021). It was a tradeoff between maintaining the body’s innate immunity (its first line of defense) and ability to degrade and deactivate the vaccine’s mRNA, and a good adaptive immune response (the second line of defense) needed if a SARS-CoV-2 infection were subsequently encountered (Parr et al., 2020; Ivanova et al., 2021; Seneff et al., 2022). Although there is still much to learn about compromised innate immunity, it has for many years been recognized as a vital part of the adaptive immune system, which is critical in responding to an infection. Dysregulated innate immune responses are considered lethal early in life and many diseases are linked to malfunction in this system (Frizinsky et al., 2019).
It was by design, that N1-methylpseudouridine, as well as lipid nanoparticles, were used by Pfizer to modify the SARS-CoV-2 mRNA. As discussed above, they were specifically used to prevent degradation of the mRNA and suppress the innate immune response (Morais et al 2021, Nance et al 2021, Wadhwa et al 2020; Borchardt et al., 2020).
Already, the approach of suppressing the innate immune response in COVID-19 vaccinees is proving problematic. Suppressing the body’s innate immune response downregulates critical systems related to cancer surveillance, infection control and cellular homeostasis (ability to maintain a steady state of chemical and physical conditions). Vaccinees are unable to upregulate their interferons (as described above) which affect numerous downstream sequences to protect the body (Pepini et al., 2017; Pardi et al., 2017; Parr et al., 2020; Liu et al., 2021).
Ivanova et al. (2021) evaluated the immune response of patients with acute COVID-19 (unvaccinated) and healthy adults after receiving the Pfizer BNT162b2 vaccine. Although infection with SARS-CoV-2 and vaccination have both been shown to stimulate an immune response, that response in the two groups was qualitatively different. In the COVID-19 patients the immune response was characterized by augmented interferon signaling and upregulation of genes associated with cytotoxicity. These responses were missing in the vaccinated group. The antibody and cellular profiles between the two groups also differed. The vaccine group elicited reduced levels of IgA and IgM antibodies compared to the COVID-19 group (Ivanova et al., 2021). This was also observed by Röltgen et al. (2022).
Another indication of impaired immune response is increased cell damage. Jain et al. (2021) reported on a study of 63 patients with “coronavirus disease 2019 vaccination-associated myocarditis (C-VAM)”. All patients were less than 21 years of age, 92% were male, all had an mRNA vaccine and except for one patient, all presented after the second dose. This is not surprising considering that Avolio et al. (2021) demonstrated that SARS-CoV-2 spike protein may prompt damage to cardiac pericytes (part of microcirculation) in vitro. The Vaccine Adverse Event Reporting System (VAERS) reported 8,090 heart disorders associated with COVID-19 vaccines in 2021 which accounts for 97.7% of all vaccine adverse events in that year (https://vaers.hhs.gov/about.html).
Degradation: Solution or Problem?
Röltgen et al. (2022) reported that they found vaccine mRNA in germinal centers (secondary lymphoid organs including lymph nodes and spleen which are important for B-cell activation) up to 2 months after a second dose. Mauger et al. (2019) also demonstrated that increased guanine-cytosine (GC) content (a feature of the Pfizer modified mRNA) as well as modified nucleotides such as N1-methylpseudouridine could extend the mRNA half-life and as a result, increase protein production.
Pfizer employed all of the known methods ((5’-cap, 5’-UTR, sequence modification, 3’-UTR and a 3’poly A tail) to prevent degradation and thereby increase the half-life of their mRNA (Mauger et al., 2019; Wadhwa et al., 2020; Nance et al., 2021). Thus, it is not surprising that clearance of the vaccine mRNA is delayed and can be found 2 months post-injection (Röltgen et al., 2022). Yet, in the Pfizer document 2.4 NONCLINICAL OVERVIEW (p. 20, https://phmpt.org/wp-content/uploads/2022/03/125742_S1_M2_24_nonclinical-overview.pdf) Pfizer states that “RNA is degraded by cellular RNases and subjected to nucleic acid metabolism. Nucleotide metabolism occurs continuously within the cell, with the nucleoside being degraded to waste products and excreted or recycled for nucleotide synthesis. Therefore, no RNA or protein metabolism or excretion studies will be conducted” (emphasis added). The modifications to the SARS-CoV-2 mRNA made by Pfizer were clearly made to prevent degradation and extend the half-life of the vaccine’s mRNA (McKernan et al., 2021; Seneff et al., 2022; Nance et al., 2021; Morais et al., 2021; Mauger et al., 2019; Svitkin et al., 2017; Kierzek et al., 2013), yet Pfizer ignored this well-established fact and contradicted its own development logic and decided that “no RNA or protein metabolism or excretion studies will be conducted” (p. 20, https://phmpt.org/wp-content/uploads/2022/03/125742_S1_M2_24_nonclinical-overview.pdf). And the FDA accepted that contradiction in Pfizer’s science.
Spike Protein Production
One final issue related to the Pfizer mRNA vaccine to be briefly mentioned here is the enhanced spike protein production, generated from the vaccine mRNA. It is included here because it is, in part, related to the use of N1-methylpseudouridine in the vaccine’s modified mRNA. There are numerous other issues, but they exceed the scope of this report. See Seneff et al. (2022) for a thorough discussion.
A side effect of N1-methylpseudouridine substitution is enhanced translation of mRNAs (enhanced protein production) (McKernan et al., 2022; Morais et al., 2021; Nance et al., 2021; Parr et al., 2020; Mauger et al 2019; Svitkin et al., 2017; Kariko et al., 2008). What problems are associated with over production of spike protein?
Brun et al. (2020) reported the process by which spike protein (S) is processed within the host cell and soluble S1 subunit is secreted into the extracellular space via lysosomes. Mishra et al. (2021) reported that excess spike protein causes microRNA (miRNA, a special type of RNA important in cellular regulatory function) to be exported out of the cells via exosomes. These released microRNAs get transported to distant tissues and organs, including the brain and central nervous system (CNS) where they are internalized and initiate a cascade of deleterious effects (Mishra et al., 2021).
MicroRNAs are being recognized as an enormously important component of gene expression and regulation and are associated with many diseases as well as immune response (O’Brien et al., 2018; Zhang et al., 2021). By the way, SARS-CoV-2 genome, including the spike protein mRNA, have been shown to encode their own miRNAs, some of which interact with human miRNAs (Liu et al., 2020). This undeniably important biomolecule was not mentioned by Pfizer either.
Conclusion
To summarize, Pfizer utilized lipid nanoparticles and a modified mRNA in which all natural uridine nucleotides were replaced with a rarely encountered nucleotide, N1-methylpseudouridine. While it solved their problems of RNA delivery, immunogenicity and degradation, it created some new problems. While uridine substitution was found to reduce the body’s immune response to the foreign RNA and protect the mRNA from degradation, there are adverse effects from this strategy.
There is practically no scientific data available on how total uridine substitution in an mRNA will affect the delicate balance of the cellular and bodily physiology of the host and what downstream effects may be initiated. Yet Pfizer conducted no studies on this issue.
Suppressing the body’s innate immune system also has downstream consequences, particularly if a SARS-CoV-2 infection is subsequently encountered. Increasing the stability and half-life of the vaccine mRNA, along with increasing its translation, means increased production of the spike protein which, as it turns out, is itself a cause of pathogenesis.
Problems with the Pfizer vaccine design and failure to adequately investigate their effects on the delicate cellular systems of the human body are already manifesting themselves. These problems are summarized in VAERS (https://vaers.hhs.gov/about.html). The long list of adverse events is a reflection of these issues.
References
Andries, O., et al., J Control Release, 2015; 217:337-344. N(1)-methylpseudouridine-incorporated mRNA outperforms pseudouridine-incorporated mRNA by providing enhanced protein expression and reduced immunogenicity in mammalian cell lines and mice.
Avolio, E., et al., Clinical Science, 2021; 135:2667-2689. The SARS-CoV-2 Spike protein disrupts human cardiac pericytes function through CD147 receptor-mediated signalling: a potential non-infective mechanism of COVID-19 microvascular disease.
Brun, J., et al., bioRxiv, https://doi.org/10.1101/2020.11.16.384594, Analysis of SARS-CoV-2 spike glycosylation reveals shedding of a vaccine candidate.
Borchardt, E., et al., Annu Rev Genet, 2020; 54:309-336. Regulation and Function of RNA Pseudouridylation in Human Cells.
Eyler, D., et al., PNAS, 2019; 116:23068-23074. Pseudouridylation of mRNA coding sequences alters translation.
Frizinsky, S., et al., Rheumatology, 2019; Nov 1;58(Suppl 6):vi1-vi8. doi: 10.1093/rheumatology/kez387. PMID: 31769855; PMCID: PMC6878844.The innate immune perspective of autoimmune and autoinflammatory conditions.
Ivanova, E., et al., 2021; Available at SSRN: https://ssrn.com/abstract=3838993 or http://dx.doi.org/10.2139/ssrn.3838993. Discrete immune response signature to SARS-CoV-2 mRNA vaccination versus infection.
Jain, S., et al., Adv Drug Deliv Rev, 2021; Dec;179:114000. doi: 10.1016/j.addr.2021.114000. Epub 2021 Oct 9. PMID: 34637846; PMCID: PMC8502079. Messenger RNA-based vaccines: Past, present, and future directions in the context of the COVID-19 pandemic.
Kang, S., Compans, R., Mol Cells, 2009; Jan 31;27(1):5-14. doi: 10.1007/s10059-009-0015-1. Epub 2009 Feb 5. PMID: 19214429; PMCID: PMC6280669. Host responses from innate to adaptive immunity after vaccination: molecular and cellular events.
Kariko, K., et al., Immunity, 2005; 23: 165-175. Suppression of RNA Recognition by Toll-like Receptors: The Impact of Nucleoside Modification and the Evolutionary Origin of RNA.
Kariko, K., et al., Mol Ther, 2008; 16(11): 1833-1840. Incorporation of Pseudouridine Into mRNA Yields Superior Nonimmunogenic Vector Increased Translational Capacity and Biological Stability.
Liu, Z., et al., arXiv, 2020; 2004.04874. Implications of the virus-encoded miRNA and host miRNA in the pathogenicity of SARS-CoV-2.
Liu, Z., et al., Journal of Biomedical Research, 2021; 35(3): 216-227. SARS-CoV-2 encoded microRNAs are involved in the process of virus infection and host immune response.
Mauger, D., et al., PNAS, 2019; 116(48): 24075-24083. mRNA structure regulates protein expression through changes in functional half-life.
McKernan et al., 2021; https://doi.org/10.31219/osf.io/bcsa6. Differences in Vaccine and SARS-CoV-2 Replication Derived mRNA: Implications for Cell Biology and Future Disease.
Morais, P., et al., Front Cell Dev Biol, 2021; November 2021;https://doi.org/10.3389/fcell.2021.789427. The Critical Contribution of Pseudouridine to mRNA COVID-19 Vaccines.
Nance, K., Meir, J., 2021; May 26;7(5):748-756. doi: 10.1021/acscentsci.1c00197. Epub 2021 Apr 6. PMID: 34075344; PMCID: PMC8043204. Modifications in an Emergency: The Role of N1- Methylpseudouridine in COVID-19 Vaccines.
O’Brien, J., et al., Front Endocrinol, 2018; 9: 402. Overview of MicroRNA Biogenesis, Mechanisms of Actions, and Circulation.
Pardi, N., et al., Nat Rev Drug Discov, 2018; 17(4): 261-279. mRNA vaccines — a new era in vaccinology.
Parr, C., et al., Nucleic Acids Res, 2020; 48(6), e35. http://doi.org/10.1093/nar/gkaa070. N1-Methylpseudouridine substitution enhances the performance of synthetic mRNA switches in cells.
Pepini, T., et al., J Immunol, 2017; 198(10): 4012-4024. Induction of an IFN-Mediated Antiviral Response by a Self-Amplifying RNA Vaccine: Implications for Vaccine Design.
Röltgen, K., et al., Cell, 2022; 185: 1025-1040. Immune imprinting, breath of variant recognition, and germinal center response in human SARS-CoV-2 infection and vaccination.
Seneff, S., et al., Food Chem Toxicol, 2022; Apr 15;164:113008. doi: 10.1016/j.fct.2022.113008. Epub ahead of print. PMID: 35436552; PMCID: PMC9012513. Innate immune suppression by SARS-CoV-2 mRNA vaccinations: The role of G-quadruplexes, exosomes, and MicroRNAs.
Svitkin, Y., et al., Nucleic Acids Res, 2017; 45(10): 6023-6036. N1-methyl-pseudouridine in mRNA enhances translation through eIF2-dependent and independent mechanisms by increasing ribosome density.
Wadhwa, A., et al., Pharmaceutics, 2020; Jan 28;12(2):102. doi: 10.3390/pharmaceutics12020102. PMID: 32013049; PMCID: PMC7076378. Opportunities and Challenges in the Delivery of mRNA-based Vaccines.
Wu, G., et al., Methods Enzymol, 2015; 560: 187-217. Pseudouridine in mRNA: Incorporation, Detection, and Recoding.
Wurm, J., et al., RNA, 2012; 18(3): 412-420. Identification of the enzyme responsible for N1-methylation of pseudouridine 54 in archaeal tRNAs.
Zhang, S., et al., Brief Bioinform, 2021; 22(2): 1137-1149. The miRNA: a small but powerful RNA for COVID-19.
Zhao, Y., et al., Front Bioeng Biotechnol, 2018; 6:8. doi: 10.3389/fbioe.2018.00008. PMID: 29473035; PMCID: PMC5809436. The Role of Noncoding RNA Pseudouridylation in Nuclear Gene Expression Events.
GREAT article! Just wonder if there is a single study out there with RNA profiling inside of the human body AFTER the mod mRNA injections??? Also is there an enzyme which possibly can demethylate that 1mp-U and make a p-U in a nonrandom fashion out of the synthetic Spike genome? That would explain why human genome editing patents, products, etc. are all in the queue….